Life span as a biomarker
Consider that living creatures, including mice and men, are assemblies of biological systems, all working together at different levels — molecular, subcellular, cellular, tissue, and organ. Life span is determined by the first biological system to fail beyond compensation. This has a critical implication for experimental design when studying basic mechanisms of aging.
We define an aging mechanism as one that coordinates the expression of diverse biomarkers of aging or that couples biomarkers of aging with life span. We do not yet know what these basic aging mechanisms are, but we do know that it ispossible to retard senescence in multiple systems simultaneously and lengthen life span. For example, when mice and rats are fed restricted amounts of food, aging mechanisms appear to be delayed and the animals live longer.
We also know that the greatest effects on aging rates appear to be genetic, as evidenced by a large variation in aging and life span among related species. For example, in the Primate order, one of the shortest-lived members is the mouse lemur, with a maximum life span of about 15 years when in human care. In contrast, it is not unusual for humans to live past 90.
Why we study maximum life span
Maximum life span is the survival statistic considered most relevant to gerontological studies because, as a cohort ages, the relative influence of senescence on the causes of mortality increases. Thus, survival data from the latter portion of the survival distribution are more likely to reflect influences of senescence than are survival data from the initial portion. Figure V.1 shows how age-independent and age-dependent risks affect total risk, and how age-dependent risk plays a greater role as an individual ages.
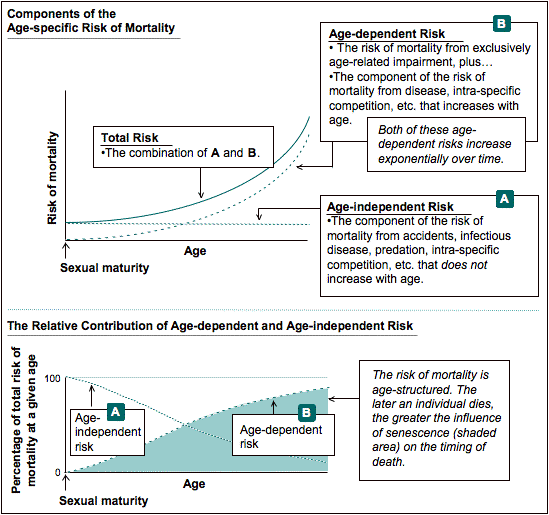
If a treatment prevents a single important cause of mortality without affecting the underlying senescence rate, that treatment is more likely to increase median life span than maximum life span. This is because the animal will die from the failure of another competing cause of mortality and will not live longer than what is normal for its cohort. On the other hand, if a treatment does retard underlying senescence rates, it has the potential to delay the expression of many age-related diseases — including those that constitute the competing causes of mortality — and, thereby, increase maximum life span for the cohort. Using a survival curve, Figure V.2 illustrates the difference between curing one disease vs. delaying aging.
Thus, although increased median life span can be an important indicator that a specific disease has been prevented, increased maximum life span is the gold standard for assays of basic mechanisms of aging.
To gather maximum life span data, we must allow animals to live as long as they can, under as little stress as possible.
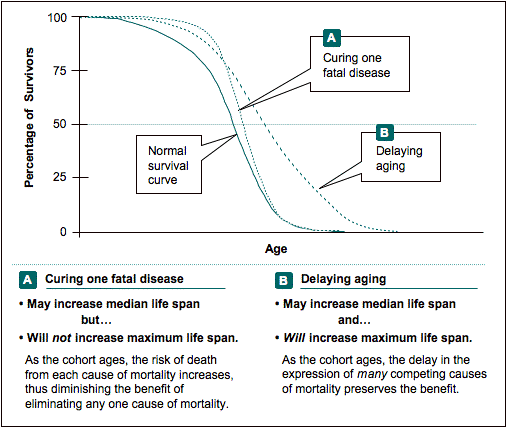
How we determine maximum life span
To get the most accurate maximum life span data possible, we must not euthanize old animals until we are certain that they would die if we did not interfere. After gaining much experience, we have determined that the following extreme symptoms (given in approximate order of importance) are the most reliable signs that a mouse will die soon:
- Unresponsiveness to touch.
- Failure to eat or drink.
- Slow respiration and cold to touch.
- Hunched up, with matted fur.
- Signs of sudden weight loss such as prominent ribs and spine; sunken hips.
Probably the most telling criterion is when an animal fails to respond to outside stimuli. But even if a mouse does not respond normally to touch, this alone does not mean that it is close to death. Some old animals are unresponsive because their senses of sight, hearing, smell, and touch have declined. In addition, they may respond much more slowly that do young mice. Thus, we check for other symptoms as well. Once we are certain that an animal will die soon, maximum life span data can be collected and the mouse can be euthanized.
In our aging colonies, we examine older mice at least 2–3 times per week. For mice with apparent health problems, we check them daily.
Why we study long-lived vs. short-lived models of aging
Short-lived models
To shorten life span, a treatment need only cause the failure of one vital biological system in the research animal. A treatment can do this easily by any one of a multitude of ways, but no aspect of shortened life span, per se, validates it as a biomarker for accelerated senescence. Validation requires the accelerated expression of other biomarkers of aging and of multiple, independent, age-related diseases. Without this validation, the likelihood is that a treatment retarded disease rather than a mechanism of aging.
Even if a treatment that shortens life span also produces multiple diseases that generate symptoms characteristic of senescence, there is no assurance that the treatment accelerates the processes that underlie the natural exponential increase in chronic disease with increasing age. A familiar example in humans is cigarette smoking, which advances the expression of some biomarkers of aging (such as diminished lung function), accelerates appearance of certain age-related diseases (such as specific cancers, heart disease, and emphysema), and often shortens life span. But cigarette smoking does not accelerate an aging mechanism. It just makes us sick. Cigarette smoking is not a valid model of accelerated aging, although it is a model of chronic poisoning.
Long-lived models
On the other hand, to lengthen life span appreciably, a treatment must delay or prevent all critical age-related diseases. This can occur in one of two ways: Either the treatment could affect each individual disease mechanism independently, implying that the treatment is a panacea, or the treatment could affect a mechanism of aging. The greater the diversity of diseases affected, the greater the possibility that the treatment alters a mechanism of aging and thus is a valid model of delayed aging.
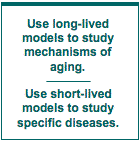
To date, only two treatments — diet restriction and the panhypopituitary dwarf mutation — have been shown to delay senescence and increase life span on a variety of long-lived genetic backgrounds in experiments replicated by independent laboratories. We consider these treatment models valid models of delayed senescence because, in unrelated genotypes that express diverse diseases of aging, the treatments delay expression of multiple biomarkers of aging and increase life span.
How we define the life history phases of mice; how they compare with humans
While aging is a continuum, for expediency, it usually is treated as a discrete rather than a continuous variable. Thus, most studies focus on two or three specific life phases: mature adult, middle age, and old. Figure V.3 illustrates these life phases using a survival curve that is based on a large cohort of C57BL/6J mice (150 males and 150 females). As a reference, we provide comparable ages for human beings and compare the maturational rates of both species.
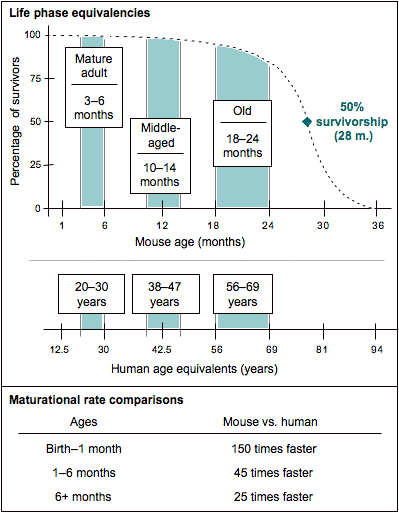
The life phases in mice
Mature adult: 3–6 months of age
The mature adult group is the reference for any age change, whether the change is developmental, maturational, or senescent. This group consists of mice that are past development but not yet affected by senescence. For the mature adult group, mice should be at least three months old because, although they are sexually mature by 35 days, relatively rapid maturational growth continues for most biological processes and structures until about three months. The upper age range for the mature adult group is typically about six months. Past this age, mice might exhibit some age-related change. (As an example, female mice are retired from breeding at eight months because litter size diminishes.) However, if a specific biomarker is known to be stable until 12 months, mice as old as 12 months may be considered mature adult.
Middle age: 10–15 months of age
Middle age refers to a phase during which senescent changes can be detected in some, but not all, biomarkers of aging. A middle-aged group is often included in studies of aging to determine if an age-related change is progressive or is first expressed only in old age. For the middle-aged group, mice should be at least 10 months old. Senescence processes that begin in younger adults (for example, collagen cross linking and accumulation of activated/memory T cells) often can be detected by then. The upper age limit for the middle-aged group is typically 14–15 months, because at this age, most biomarkers still have not changed to their full extent, and some have not yet started changing.
Old: 18–24 months of age (or older, depending on genotype)
Old age refers to a period when senescent changes can be detected in almost all biomarkers in all animals. For the old group, mice should be at least 18 months old. For C57BL/6J mice and many other genotypes, the upper limit is 24 months, but this depends on the onset of disease for the specific genotype.
Genotype differences
Age ranges for young adult and middle-aged groups are consistent among most inbred strains and F1 hybrids. However, the upper boundary for the old groups, 18–24 months, should be adjusted for each genotype based on relative survivorship and age-specific incidence of disease. A general guideline for the upper age limit for biomarker studies in any genotype is the age at 85% survivorship, unless mice are necropsied.
Even at 18 months of age, in many strains of mice, misleading results may be produced by diseases that affect biomarkers, so necropsies are very useful. In general, as the mice approach and go beyond the median life span for their genotype, necropsies become increasingly important to determine whether diseases have developed that may affect the study. With necropsies, the oldest mice can be used to study terminal processes of senescence and disease.
Note: Some of the material in this page was taken from: Flurkey K, Currer JM, Harrison DE. 2007. The Mouse in Aging Research. In The Mouse in Biomedical Research 2nd Edition. Fox JG, et al, editors. American College Laboratory Animal Medicine (Elsevier), Burlington, MA. pp. 637–672.