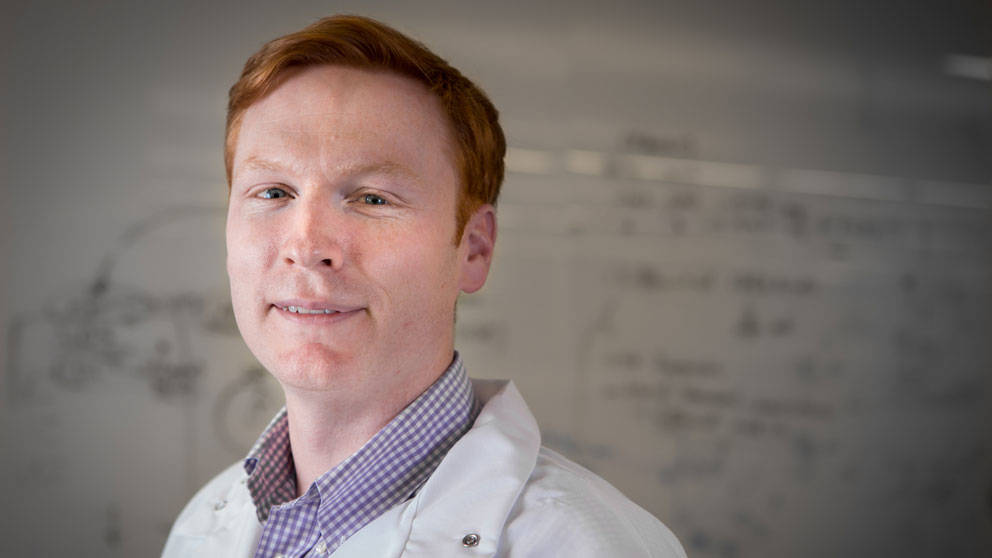
Travis Hinson, M.D. JAX photo by Tiffany Laufer.
The regeneration of tissues is routine for some vertebrates, but is lacking for most human tissues. The lack of regeneration has particular relevance to the human heart. Damage from insults like heart attacks frequently results in heart failure and death, and better medical options are needed. A major question in heart research is why our damaged hearts cannot repair themselves unlike a zebrafish’s heart, which regains full function if injured. If we can discover exactly why human heart tissue does not regenerate, it could open an exciting new avenue for cardiovascular therapy development.
Animal model research has provided many clues, but there are important differences between human cardiomyocytes—the cells that contract in the heart—and those from other organisms, even other mammals. A team led by Jackson Laboratory (JAX) Assistant Professor J. Travis Hinson, M.D.Utilizes genomic approaches like CRISPR/CAS to interrogate mechanisms of inherited cardiovascular disorders especially those that lead to heart failure.J. Travis Hinson, M.D., who has a joint appointment with UConn Health, is therefore using the latest cellular technologies to engineer human cells to investigate the molecular mechanisms preventing human heart regeneration. In “Sarcomere function activates a p53-dependent DNA damage response that promotes polyploidization and limits in vivo cell engraftment,” published in Cell Reports, Hinson presents new findings about what blocks cell division and renewal in adult human heart cells.
A key characteristic of mature human cardiomyocytes is that they become mononuclear polyploid before adolescence. What that means is that, like most other cells, they only have one nucleus, but they have more than the usual two chromosome copies per cell. Indeed, the DNA content per cardiomyocyte has been found to increase 1.7-fold, indicating that while the cell division cycle may start—and the chromosomes duplicated—it is not completed for reasons not completely understood. Obtaining viable heart cells from living humans is not feasible because biopsies are associated with high complication rates, so Hinson engineered human induced pluripotent stem cells (iPSCs) to produce cardiomyocytes that could be tracked through the cell division cycle. Over time, they displayed progressive polyploidization and became unable to replicate, properties similar to those seen in the heart of an eight-year-old.
Through using a genome-wide screen based on CRISPR technology, Hinson identified p53 as a key part of the process. Well known as a regulator of cell division and tumor suppressor, mutations in p53 underlie many cancers and their uncontrolled growth. In cardiomyocytes, however, the researchers found that the high-energy function of sarcomeres—the contractile structures—yields reactive oxygen species that promote a DNA damage response. This in turn activates p53 to inhibit cyclin B1, a vital switch in the cell division cycle. The result is that cell division is blocked in a process similar to that of p53’s tumor suppressor function. In other words, the normal cardiomyocyte function—contraction—induces a signaling pathway that effectively stops the completion of cell division relatively early in our maturation. While the cardiomyocytes continue to work with remarkable endurance and durability, in humans they are mostly locked in for life and unable to regenerate if damaged.
Can this finding have implications for human heart regeneration? To find out, Hinson studied an animal model of heart attacks into which human cardiomyocytes are transplanted to restore function. Hinson postulated that by reducing sarcomere function the reduction in p53 could improve cell engraftment rates. Indeed, the injected cardiomyocytes with inhibited sarcomere assembly exhibited significantly higher engraftment and remuscularization rates than the normal wild-type cells due to increased replication, indicating that they had relatively higher regenerative capacity. The results indicate that inhibiting sarcomere function or p53 could provide a lever to enhance cardiomyocyte cell therapy for treating patients with heart attacks.