Addiction researcher Vivek Kumar
By blending classical and cutting-edge genetic approaches, Vivek Kumar is unveiling the genes at work within the brain to control complex behaviors, such as anxiety and addiction.
The brain is the most complex system in the body. It is home to billions of neurons, which mingle with other cells, making trillions of minuscule connections. This delicate circuitry forms the ultimate command center, controlling our thoughts, actions and emotions. Deciphering its structure and its major outputs — chief among them, behavior — is one of the most important and perplexing frontiers in modern science.
Assistant Professor Vivek Kumar is one of the fearless explorers of this uncharted world. He joined The Jackson Laboratory (JAX) in early 2015, launching a research group devoted to some of the most complex, yet profound, questions in neurobiology: What is the biological basis of complex behaviors, such as addiction, anxiety and depression? What genes are involved? And how do mutations — changes in the genetic code that can alter how and when these and other genes act — alter the cellular form and function of the brain?
These are difficult questions to ask and answer, but Kumar is hardly one to wither when the going gets tough. “He’s not afraid to take on a new challenge — to change and learn something completely new,” says Joseph Takahashi, a professor and chair of the department of neuroscience at the University of Texas Southwestern Medical Center, and one of Kumar’s mentors. “You have to be able to move into different fields and technologies to really make new discoveries, and Vivek is able to do that.”
Kumar, along with Assistant Professor Elissa Chessler, Ph.D., are now sharing their passion to solve these complex questions in neurobiology through a newly created educational program at JAX titled the Short Course on the Genetics of Addiction. This unique course brings together world-renowned experts in addiction, human genetics, and mouse genetics. Kumar and Chessler are focused on addressing the current addiction epidemic and designed the course for students of all experience levels who seek an introduction to the field and desire to hone their genetic skills and knowledge. Their hope is attendees will learn the latest tools and approaches in addiction and genetic research to return to their own communities and make meaningful contributions to science and society.
The catalyst
Kumar was born in India and arrived in the U.S. when he was nine years old. Growing up in Memphis, Tenn., his parents had visions of him becoming a doctor. Although he was not strictly opposed to the idea, Kumar got swept up in the excitement of laboratory research at a young age. He remembers vividly his earliest experiences in science, especially participating in his high school science fair: His first time out, he won a $25 prize, courtesy of the U.S. Army.
“That prize was a real motivator,” recalls Kumar. “For the next year’s science fair, I contacted a faculty member at the University of Memphis, and she allowed me to come into her lab and do a more traditional research project, focused on a scientific question.”
His question centered on the interactions between plants and bacteria, specifically how bacteria colonize the roots of legumes in a process known as nodulation, and the molecular signals that are at play. This time, Kumar’s work earned him a trip to the Memphis regional science fair, and eventually, a spot in the international science fair, held that year in Tulsa, Okla.
“I got to meet all these science geeks from all over the world,” he says. “That was the catalyst. It inspired me to approach things in a scientific way.”
Once-in-a-lifetime opportunity
After earning his undergraduate degree from the University of Chicago, Kumar headed west for graduate school. At the University of California, San Diego, he worked in the laboratory of Michael Rosenfeld, where he spent six years dissecting a key protein involved in transcription — a fundamental activity cells use to convert (or “transcribe”) the information within DNA into a more nimble chemical form, made of the related genetic material, RNA.
“My graduate career focused on hard-core biochemistry and structural biology approaches, and [as I considered my next step], I felt I was really lacking a genetic component,” says Kumar.
He learned that Takahashi, then at Northwestern University, had received a major grant to apply genetic approaches in mice to unlock the basis of complex behaviors, such as learning, anxiety, impulsivity and addiction. In the 1990s, Takahashi pioneered the discovery of a critical gene in the mouse, called Clock, which is now recognized as the molecular mastermind of circadian rhythms —biological processes, such as sleep, that fluctuate on a 24-hour cycle.
Takahashi’s find was remarkable for two reasons. First, Clock was the first gene to be linked with circadian rhythms in mammals, opening up the field of circadian biology. Second, it was identified through a classic “forward genetics” approach, in which disease models are created (using methods that can perturb the genome, such as chemicals or irradiation), analyzed for unusual biological traits (called phenotypes), and then meticulously studied at the DNA level to pinpoint the culprit gene mutation.
Historically, such forward genetic screens have been a mainstay of research in the fruit fly, Drosophila melanogaster. The notion of applying them in mice — and to an area as complex as behavior — was seen as a bold move. Bolstered by Clock’s discovery, Takahashi subsequently launched a five-year, large-scale, forward genetics screen in mice to uncover genes that affect other complex behaviors.
While interviewing Kumar for a postdoctoral position, Takahashi told him that if he came to the lab, he could lead the portion of the screen devoted to cocaine response. “I have to be honest; I didn’t know that much about the screen at the time, and I certainly had never worked in mice,” says Kumar. “But it was one of those once-in-a-lifetime opportunities.”
Kumar was hooked.
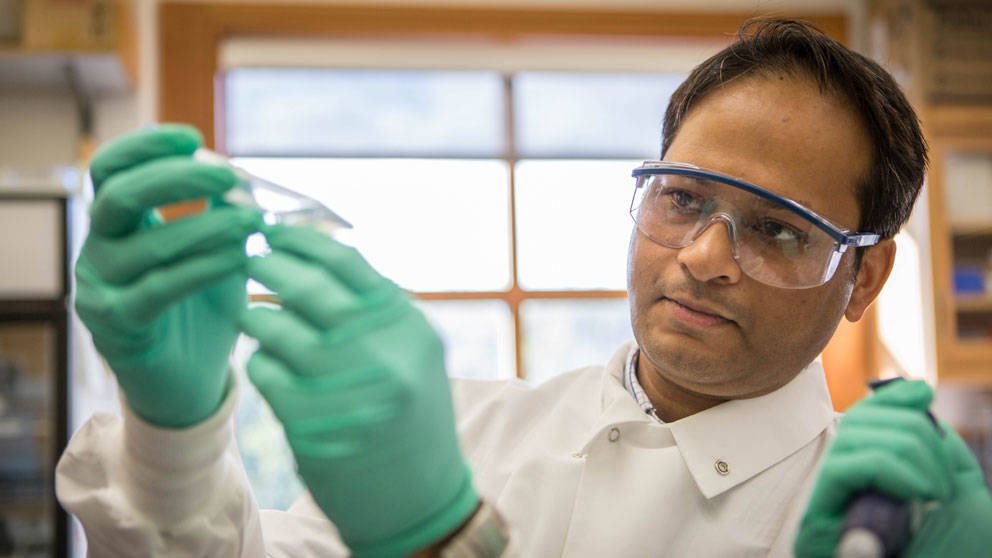
From mice to maps
As he settled into Takahashi’s lab, Kumar set to work on his screen. The goal was to identify mice with unusual responses to a dose of cocaine. Typically, after receiving an injection of cocaine, mice become more active, running around their cages more quickly and for longer periods than in the absence of the drug.
Ideally, he would uncover mice whose behavior diverged significantly from that norm — so-called six standard deviation mutants, like Clock. These extreme outliers are not only easier to discern, but they also lend themselves more readily to genetic analysis. That means their behavioral defects can often be traced to a single errant gene. “Our mind was set on finding these types of mutant mice, but it just was not the case.”
Two years and some 20,000 mice later, the mouse mutants in Kumar’s collection were disappointingly mild, just one to one-and-a-half standard deviations. With such subtle effects, the standard tools for mapping gene mutations simply would not work. “I realized I needed more skills — I wasn’t going to cut it with traditional mapping approaches,” recalls Kumar. “I needed to learn statistical genetics.”
To step up his genetic mapping game, Kumar came to JAX, spending a week in Bar Harbor as a student in Gary Churchill's course on systems genetics.
“The knowledge I gained through his course gave me the tools to go back to the lab and start thinking about ways of mapping my mutant mice,” says Kumar.
Serendipity
Although Kumar’s newfound knowledge was a boon, it did not change one of the stark realities of statistical genetics approaches. The portions of the genome that emerge through the mapping process tend to be quite large, leaving a sizable amount of genomic real estate that must be searched to find the offending genetic change. In the absence of known genes that can serve as likely suspects and thus help narrow the search, the task can be a major challenge.
But thanks to the power of next-generation technologies for decoding DNA, this once-huge hurdle — whittling down 50 million or so genetic letters to a single discrete typo — has now been reduced to a speed bump.
As Kumar prepared to map his stable of mutant mice, 10 of them in total, he searched for a suitable mouse strain to mate with them. (These so-called mapping crosses are a key first step in the mapping process.) He examined several different strains, including the well-known strain, C57BL/6 (called “black 6” for short), which comes in two seemingly identical varieties: C57BL/6N and C57BL/6J.
Through this process, he stumbled upon a startling discovery. “I found that the black 6N had a different cocaine response than the black 6J, and that was really surprising because these were considered to be essentially the same stock of mice.”
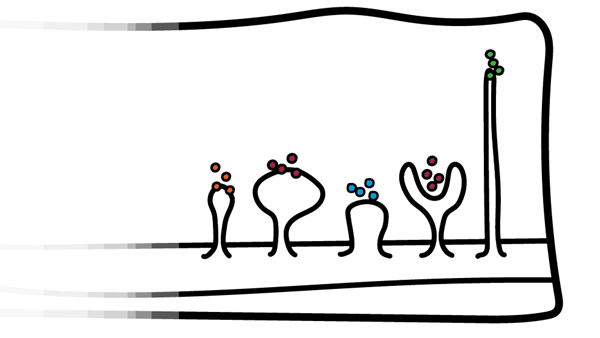
First established by C.C. Little, the founder of The Jackson Laboratory, the black 6J have been maintained in Bar Harbor for decades. In 1951, a colony was shipped to the National Institutes of Health, establishing a second source of the mice, known as black 6N. Although researchers often use these mice interchangeably, Kumar’s finding hinted at an important difference — one that led him directly to a gene that is mutated exclusively in the N sub-line and causes a diminished cocaine response.
In a paper published in Science in late 2013, Kumar reported that mice carrying mutations in this gene, called Cyfip2, have abnormal neuronal structure in a key part of the brain known for its role in behaviors, including addiction. These neurons have fewer hair-like projects (called dendritic spines), which are important sites of neurochemical signaling.
The serendipity of this Cyfip2 find is not lost on Kumar. “In one case, we were creating mutations using a chemical, and in the other, there were 50 years of genetic drift. The end result was the same — a single nucleotide change causes a large behavioral difference.”
Peering into the treasure chest
Now, as he establishes his own laboratory at JAX, Kumar is extending his work on Cyfip2. One major project is to unravel the biology of Cyfip2 and understand its potential role in addiction, using specialized behavioral tests. On a broader level, his lab will focus on how the circuit that regulates motivated behavior is constructed and maintained at the molecular level, using Cyfip2 as an entry point. His group will also explore how changes in neuronal shape (known as structural plasticity) affect drug responses. Through these experiments, Kumar and his team can determine whether mutations in Cyfip2 are connected with voluntary cocaine intake.
He also has his collection of mutant mice from the screen in Takahashi’s lab. Kumar has already genetically mapped some of them, and now the precise gene mutations must be found. The behavioral defects in these mice span not just addictive behaviors, but also anxiety and hyperactivity, offering biological inroads into a range of complex disorders in humans — from addiction to depression to attention deficit and hyperactivity disorder (ADHD).
“Not many people are willing to take this approach. And because Vivek has a bank of mutant mice that he’s already found, it’s like a little treasure chest — he can go in there and keep finding new genes,” says Takahashi.
Perhaps more than anything else, Kumar views his mutant mice as a means to decipher a fundamental part of the brain, called the mesolimbic pathway. This area connects multiple neuroanatomical regions and is driven by the chemical signal, dopamine. Also known as the reward pathway, it plays a crucial role in impulse control and motivation.
“This circuitry is really a survival mechanism,” explains Kumar. “Every day, an animal has to make a decision whether it is going to stay safe in its nest or go out and explore — find food, a new mate, a better place to live. It is this delicate balance that the [mesolimbic] circuitry regulates and that drugs of abuse hijack.”
He also hopes to steer his laboratory in another important direction: developing a new generation of mouse neurobehavioral assays. Many of the existing tests of mouse behavior are highly artificial and do not adequately mimic an animal’s natural activities. That has significant implications for the genes that can be identified through forward genetic screens, which rely on these behavioral tests as the primary means to detect mutants. “Going forward, I think we’ll need a melding of the minds from folks from computation, engineering and genetics,” says Kumar. “This field, known as computational ethology, is in its infancy and holds great promise.”
With innovative new tests that are more robust and more scalable, a new universe of genes could be revealed. Kumar believes JAX is the perfect place for him to pursue this work.
“JAX has such a great history,” says Kumar. “I’m really thankful to have the opportunity to contribute to this organization, and I look forward to what we together — my lab and JAX as a whole — can accomplish.”
Nicole Davis, Ph.D., is a freelance writer and communications consultant specializing in biomedicine and biotechnology. She has worked as a science communications professional for nearly a decade and earned her Ph.D. studying genetics at Harvard University.