Researchers are able to do many things today that were impossible five, three, even one year ago. Some of the new options are science-fiction-level impressive. Others seem somewhat mundane but carry profound implications for research. All of them provide an important step forward, and researchers are able to manipulate and understand biology with unprecedented detail and accuracy.
There are many possible methods and capabilities to include, but this series will focus on:
- Improved single-cell methods
- Advances in long-read sequencing and analysis
- Genetic engineering – CRISPR/cas9 and beyond
- Microbiomics
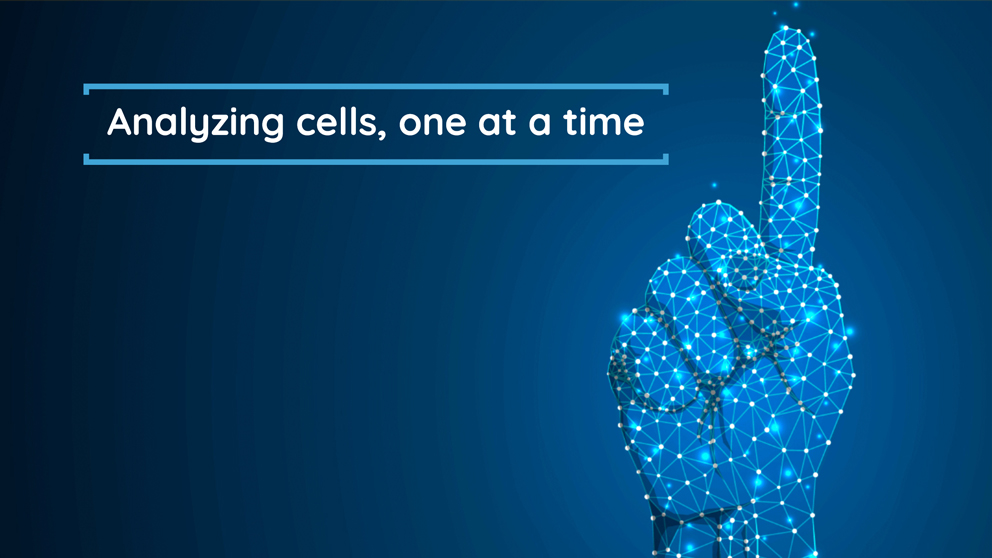
Improved single-cell methods
For many years, every reference to cellular traits, such as genome sequencing or RNA sequencing, was based on a mashup of millions of cells. That many were needed to acquire sufficient material with which to work. The results captured the dominant cell type within the sample, but important differences between individual cells were lost. In a way it has mirrored medical practice—precision medicine seeks to tailor medical care to individuals rather than relying on population averages, so characterizing individual differences is essential.
The past few years have seen the rapid development and deployment of single-cell methods, however. It’s now readily possible to generate DNA sequences and transcriptomes (all the messenger RNA present in a cell) as well as protein and metabolite data on a cell-by-cell basis. Some fields, such as cancer, are obvious beneficiaries, as it’s been recognized for a while that tumors are highly heterogeneous, meaning they are composed of many different cellular subtypes. But single-cell methods have also been revealing far more about our overall biology. There are many examples, but important ones include the discovery of more cell types than previously recognized within different tissues, Exploring the hypothalamus one neuron at a timeA collaborative team from UConn and The Jackson Laboratory have characterized neuron diversity in the lateral hypothalamic area (LHA), a critical coordinator of fundamental behaviors in the brain. The results provide important clues for understanding LHA neural circuits and the potential development of targeted neuropsychiatric therapies. including the brain , and the fact that every person is a mosaic, meaning there are genomic differences among cells within an individual, some of which may be important.
Returning to cancer, single-cell methods make possible a detailed assessment of cancer cell evolution under stress, such as from chemotherapy. Rapid evolution underlies cancer recurrence and spread, and while treatment may eliminate most cells, sub-populations that evolve to be therapy-resistant may survive and expand. The result is new tumors and a far worse patient prognosis. Research into how the cells evolve and the resistance mechanisms they are most likely to acquire can inform front-line therapy, such as combination therapies that address both the up-front pathways and those predicted to develop.
Other applications also have important implications for both normal biological function and disease. For example, the cells in pancreatic islets play crucial roles in blood glucose regulation and diabetes. Researchers have devoted much of their attention to pancreatic beta cells, which is understandable because they are the most common cell type and because they secrete insulin. But there are several other cell types present, from alpha to gamma, including the rare (<1%) epsilon cells. Researchers used single-cell methods to distinguish among the cell types and looked at gene expression differences between them. Interestingly, delta cells, which comprise about 10% of the islet cells, exclusively expressed genes previously linked to type 2 diabetes. According to JAX Assistant Professor Michael Stitzel, Ph.D.(De)coding the regulatory landscape of human pancreatic islets and other metabolic cell types health and diabetesMichael Stitzel, Ph.D., it appears that the delta cells and other rare cell types may direct the islet’s function and play crucial roles in the development of diabetes.
Recent progress has enabled researchers to apply several single-cell methods at the same time. Why is that useful? One example is the fact that each gene can give rise to several different protein forms, known as isoforms, because of differences in messenger RNA (mRNA) processing within the cells. Every mRNA has additional sequences that are spliced out before it goes to the ribosome for translation to a protein. Dysfunction in the process can contribute to disease, but it’s impossible to identify from sequences alone. The ability to sequence DNA and RNA and characterize the proteins simultaneously within a single cell is an important step forward. JAX Assistant Professor Zhengqing Ouyang, Ph.D., helped Revealing cellular traits, one cell at a timeScientists have developed an expanded toolkit that enables investigations of cellular traits at the single cell level at an unprecedented level of detail. develop a toolkit, called ECCITE-seq , that gives researchers the ability to detect splicing errors leading to protein isoforms that contribute to disease.
What does the future hold? One obvious focus is the Human Cell Atlas, an international effort “to create comprehensive reference maps of all human cells … as a basis for both understanding human health and diagnosing, monitoring, and treating disease.” Mapping all the cells in the human body is a massive undertaking, and it will take the development of even more powerful, accurate and high-throughput single-cell methods to succeed.
Within the cells themselves, researchers are looking to delve into genomics beyond sequence. The three-dimensional configuration of chromatin, the highly structured combination of DNA and histone proteins around which it’s wound, is an important component of gene expression and regulation. Now JAX’s who directs the genome technologies group, is looking to characterize 3D genome structure in single nuclei, with single-molecule resolution. Preliminary results are promising, and successful implementation of the technology has, says Wei, the potential to “revolutionize the field of 3D genome biology.”