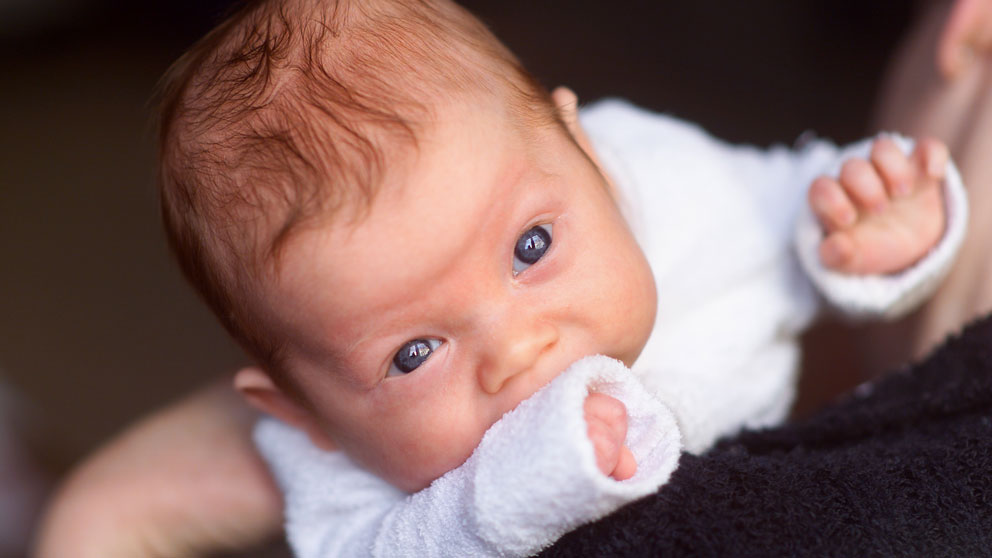
“Germs,” the invisible carriers of disease and infection, have long had a bad reputation. But we now know that we co-exist with trillions of them, also known as our microbiome — the community of bacteria, viruses, fungi and archeae that live on and within us — in relative harmony. In fact, the relationship is largely symbiotic, or mutually beneficial. Bacteria in our gut help us absorb vitamin K and break down otherwise indigestible carbohydrates, and bacteria in our skin and nose block pathogenic species from colonizing us and speed up the healing process when the skin barrier is breached. In return, we provide them a safe haven, with adequate food and a temperature-controlled environment.
But where do a baby’s first microbes come from and what controls which bugs stick around? As I have covered previously, the adult microbiome is largely stable over time, both on the skin and in the gut. Babies and children, however, are a different story. The changes that occur in their microbial communities and how and when they become colonized by different microbes (a.k.a. microbiome acquisition) are important issues and the subject of intensive study. Some scientists hypothesize that how and when the microbiome interacts with the immune system, for example, may influence some long-term health outcomes. Knowledge gained in this area will help bridge some of the gaps that are hindering our ability to fully understand our microbiome, how it influences health and disease, and how it might be leveraged for medical intervention.
In utero
The story begins earlier than previously thought. The developing fetus is inoculated with bacteria long before its birthday, contrary to about 100 years of scientific belief that the womb is a sterile environment. Microbes can be found in the placenta, umbilical cord, and amniotic fluid. The community of microbes found in the placenta is most similar to — yet unique from — the oral microbiome of adults, indicating that there may be active recruitment of certain microbes to the uterus. This study was also able to group the placental microbiomes of babies born pre-term from those born full-term, which suggests that the placenta is acquiring different microbes throughout gestation. Another study found that the average fat content of the maternal diet during pregnancy influences the microbes present in a baby’s first bowel movement, indicating that the gut may be colonized before birth.
A grand entrance
The birth canal is another early source of microbes, and several studies have found differences between the microbiomes of babies born vaginally or via caesarean section (C-section). However, while the two groups do segregate, the variability in the microbiomes from the vaginal group results in overlap with the more tightly grouped C-section babies 6 weeks after birth. And there is still not enough research to determine whether these differences are important for long-term health. To my knowledge, for instance, no one has tried to parse adult microbiomes based on mode of delivery as an infant, likely because the influence of diet and environment are too strong. Similarly, while there are several studies performing correlative analyses that link C-section to allergies and immune dysfunction, it is very difficult to segregate these factors from environment. For example, socio-economic status (SES) correlates with C-section rates, wherein women with the lowest SES are more likely to have a C-section in developed countries. Current hypotheses as to why this disparity exists often cite this population’s decreased access to prenatal care and proper nutrition. Additionally, income disparity substantially influences the post-natal environment. For instance, lower SES women are less likely to exclusively breastfeed, which has a demonstrable impact on the baby’s early microbiome as well (see below). Thus, it is difficult to parse the independent influence of vaginal delivery on the microbiome and whether it alone skews children away from diseases like asthma. Some researchers have shown that wiping C-sectioned babies immediately post-delivery with vaginal secretions from the mother overcomes initial community differences between vaginally- and C-section-delivered babies. However, whether this post-C-section vaginal colonization reduces the risks for asthma and auto-immune disease has yet to be determined.
Diet
The influence of diet on the gut microbiome is arguably the strongest of any external factor. Therefore, it is not surprising to learn that babies who receive formula only have a very different gut community than those solely breastfed (babies fed a combination of both cluster in between). But again, there are added layers of complexity. For one, breastfed infants are more likely to be routinely exposed to mother’s skin microbiome than exclusively formula fed infants, which likely influences the developing gut community. Additionally, the nutritional components of breast milk and formula are very different. For instance, the predominate protein source in human milk is whey, but the predominant protein source in standard formula is casein. Therefore, the gut community of a breastfed baby might have a higher abundance of bacteria specialized at metabolizing whey than a formula fed baby.
Importantly, there is evidence that human breast milk is specifically engineered to promote the growth of certain bacteria in the infant gut. Every mammalian mother produces complex sugars called oligosaccharides, but humans pump out an abnormally high variety into their milk; scientists have identified more than two hundred human milk oligosaccharides (HMOs) to date. They are the third-most plentiful ingredients in human milk, after lactose and fats, but babies cannot digest them. Why, then, do human mothers expend all that energy to make such a diverse collection of sugars? HMOs pass intact through baby’s small intestine to the distal gut where most of our gut microbes live. HMOs are believed to be bacteria food, not baby food.
In support of this idea, many HMOs are preferentially digested by Bifidobacteria (a common component of probiotic yogurt and capsules) and Bacteriodes, and breastfed babies have a higher abundance (more than two-fold) of Bifidobacteria than formula fed babies. To determine what, if any, benefit this might have to the growing infant, researchers looked at the secretion of HMOs in breastfeeding African mothers, and found that the abundance of certain HMOs in breast milk correlated with decreased rates of infant mortality, improved body weight-by-age, and growth promotion. This suggests that some HMOs may feed bacteria that can metabolically protect infants from under and mal-nourishment, and gets closer to causal relationships between HMOs, the microbiome, and positive outcomes in infants.
Environment
Geographic microbiome differences are likely shaped by factors such as climate differences that alter the soil microbiome, genetic differences that may influence microbial acquisition, diet, and cultural practices. As an example, one study found children raised in rural Burkina Faso, Africa had guts dominated by Bacteroidetes bacteria, namely Prevotella, while children raised in urban Florence, Italy were dominated by Firmicutes bacteria. Whether these differences affect the health or fitness of these children is unknown.
Antibiotic exposure
In the developed world, antibiotic usage in early childhood is common: In the U.S., children are given an average of two courses of antibiotics before their second birthday, and that average increases with every year of life. And while antibiotics are often necessary, life-saving drugs, most kill both good (normal gut) and bad (infectious) bacteria. Decreased bacterial diversity (i.e., unique bacterial groups) in the gut and on the skin is associated with many illnesses and negative health outcomes. Researchers have shown that antibiotic usage reduces the number of unique bacteria in the gut of children two and under. Further studies are needed to determine how these disturbances influence the long-term health of antibiotic-exposed children and whether restoration of the diversity of the community post-antibiotic treatment might protect from negative health outcomes.
Caveats
Overall, longitudinal studies demonstrate that gut microbiomes begin to stabilize around 1-2 years old. The skin microbiome stabilizes post-puberty. However, it is important to note that there are gaping holes in our understanding and major questions still to address.
First, what we know about our microbial partners covers just a fraction of what actually lives with us. Bacteria are far and away our most studied partners, yet they are not alone, and they are not even the most abundant members all the time. As I mentioned previously, viruses, fungi and Archeae also colonize us, and their importance to our overall health are only now being realized. Second, while bacteria are the best studied microbes, current analysis techniques can still only identify a small proportion of them. That is because microbiomics best categorizes what it has seen before. As Julia Oh, Ph.D., assistant professor at The Jackson Laboratory for Genomic Medicine puts it, somewhere around 40-90% of the microbial content on the skin is “dark matter” (i.e., unidentifiable) and therefore difficult to study. And even abundant bacteria can pose a challenge. Prevotella is a very common member of the gut community, and its abundance in the gut has been linked to a variety of illnesses including arthritis and obesity in Western cultures. Yet, because it’s exceedingly difficult to grow in the laboratory, we understand very little about its biology. Lauren Petersen, Ph.D., a post-doctoral associate in the laboratory of George Weinstock at JAX Genomic Medicine, is working to culture Prevotella from stool. She has linked its abundance to improved athletic performance and is actively pursuing a deeper understanding of how this bug affects nutrient uptake in our gut.
Finally, because longitudinal human studies are challenging, and because humans interact daily with a constantly changing environment, human microbiome studies are largely correlative. In other words, we can identify differences in microbial communities between diabetics and non-diabetics for example, but whether those differences are a result of the disease or actually influence disease incidence or manifestation are conclusions that remain largely out of reach. As such, when an interesting correlation between microbes and disease is made in humans, many researchers attempt to recreate the phenotype in mice. Mice are an excellent surrogate for human microbiome studies because their genetics, diet, and environment can be totally controlled, thus eliminating many of the confounding variables present in humans. Additionally, mice can be born “germ-free,” which gives researchers the power to study microbes of interest in the absence of other, potentially confounding, microbes. Moving forward, increasingly complex mouse models of human microbiome studies will remain critical to pushing microbiome research from a correlative to a causative space, where we can begin to make good on these associations in clinical practice.